3D MICROSTRUCTURES FABRICATION OF
PARAFFIN OR PLASTICS WITH LASER HEATING
Keywords: Microfabrication, Microstructures, Laser heating, Paraffin, Thermoplastic materials
Abstract
This page describes a novel fabrication technology for three dimensional (3D) microstructures of thermoplastic materials with laser heating. This technology employs the phenomenon that a thermoplastic material melted by laser heating grows into a convex shape with a high aspect ratio in a liquid. It does not require a mask or mold, therefore, the microstructure can be manufactured in a short time with a low cost. The size of the microstructures can be controlled by parameters of laser irradiation, including power and time. With this technique, we have fabricated various microstructures such as pillars, oblique pillars, and lines from paraffin. Also, a microstructure of polyethylene, one of general-purpose plastic materials, has been fabricated.
Introduction
Recently, 3D microstructures have been used for fluidic, electronic, optic, and mechanical devices in various fields. Especially in the fields of chemistry and biology, there are many studies using microstructures such as synthesis of chemicals, cell cultivation, and DNA separation. Most of those microstructures are fabricated by conventional lithographic process, LIGA, and LIGA-like process. For example, a poly(dimethylsiloxane) (PDMS) microfluidic device, which is useful for chemical or biological experiments, is formed by molding PDMS prepolymer to the SU-8 master produced by lithography and bonding the replica on the cover plate. These processes require complicated steps to fabricate microstructures, including masking, molding, and bonding. Therefore, there is much demand for 3D microfabrication techniques without lithographic process for faster and cheaper manufacturing.
One of the simplest fabrication processes is to fabricate microstructures in a sealed space. Micro stereolithography is an effective technique to achieve that purpose because microstructures are obtained by light-induced polymerization of a liquid resin in this technique. Stimuli-responsive hydrogels allow in-situ fabrication of microstructures in a microchannel. However, these techniques have a limitation on materials that can be used for producing microstructures. In a biological application, the influence of these materials on cells or other organisms must be investigated.
In this page, we describe a novel technology to realize 3D microstructuring of paraffin and thermoplastic materials which are generally used in chemical and biological experiment. This technology does not require the complicated steps to fabricate 3D microstructures. It can also realize an in-situ fabrication of microstructures in a sealed space filled with liquid.
Fabrication concept
Basic concept
The technology we developed employs an interesting phenomenon that a thermoplastic material melted by laser heating grows into a shape with a high aspect ratio in a liquid. This phenomenon is caused by thermal expansion and heat release to the liquid. Figure 1 illustrates a schematic view of the formation process of 3D microstructure. A material in a liquid is locally melted and fluidized by laser heating (a). Because the liquid is transparent at the wavelength of the laser, laser is absorbed at and beneath the surface of the material. The fluidized portion of the material grows into a convex shape in the liquid (b, c). The molten material solidifies immediately after the laser irradiation is switched off because the heat of the molten material diffuses into the liquid instantaneously (d).
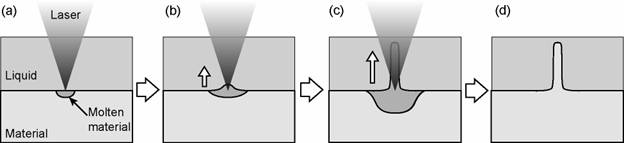
Figure 1: Schematic view of the formation process of 3D microstructure.
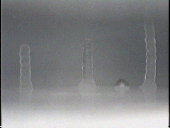
Movie (Mpeg1, 1.32MB, this is free to download):
(Formation of paraffin pillar in water. Laser power: 5.0 W. Irradiation time: 3.0 s.)
Advanced fabrication
Figure 2 shows the conceptual diagrams of fabricating microstructures by this process. Microstructures with dot or pillar shape are available by perpendicular laser irradiation without scanning (a). Structures of higher complexity can be fabricated by various methods of laser irradiation; inclined laser irradiation allows fabrication of oblique microstructures (b), scanned laser can make lines or walls (c). The size of microstructures depends on the power density of the laser, laser irradiation time, laser scanning velocity, and the temperature of the material and liquid.
If a cover plate, transparent to the wavelength of laser, is put on the liquid, the fabricated structures will bond to this cover as shown in Figure 2(d, e). The space enclosed by a continuous wall and the cover can be used as a micro chamber which is isolated from its circumference (d). The space enclosed by two walls and the cover can be used as a micro channel (e).
This process has two main advantages. It does not require the bonding process. It can also realize an in-situ fabrication of additive microstructures, shown in Figure 2, in a sealed space filled with liquid.
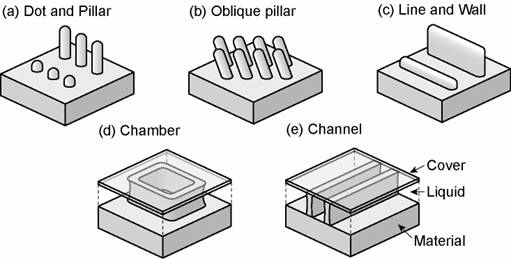
Figure 2: Conceptual diagrams of microstructures fabricated by our technique.
(a) Dot and pillar. (b) Oblique pillar. (c) Line and wall. (d) Micro chamber. (e) Micro channel.
Experiment
Experimental setup
Figure 3 illustrates a schematic view of the experimental setup. This setup consists of laser, optical components, stage, and temperature controlling device. A diode-pumped Nd:YAG laser operating at a wavelength of 1064nm is used as the light source. The laser oscillation is in a continuous wave mode. The circular Gaussian beam profile has an estimated beam propagation factor M2 = 1.02 and an initial beam diameter of 0.80 mm at 1/e2 of the peak intensity. The power of the laser is 10 W in its maximum and can be reduced by a half-wave plate and a polarizing beamsplitter. The laser beam is focused by a lens with the focal length of 30mm. The calculated values of diameter of the laser focus and the Rayleigh length of the focused laser beam are approximately 38 µm and 1.1mm, respectively. A surface image of the object is acquired by a charged-coupled device (CCD) camera.
An x-y-z stage translates a target object in three degrees of freedom. The x-y axis is used for laser scanning and the z axis is used for controlling the focal position of the laser. A tilt stage is employed for adjusting the angle of laser irradiation to the object. The laser is refracted at the surface of the liquid on the material as shown in Figure 4.
A peltiert device and micro ceramic heater are utilized for controlling the temperature of paraffin and polyethylene plate, respectively. These devices are situated under the materials as shown in Figure 3 and thermally connected with the materials by silicone grease.
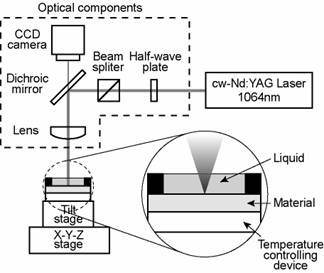
Figure 3: Schematic view of the experimental setup.
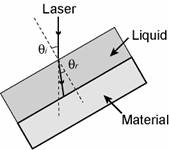
Figure 4: Refraction of laser at the surface of liquid.
Materials for fabrication
Figure 5 shows the two materials that are used in the experiment. Figure 5(a) is paraffin (melting point: 60-62oC) which contains 10% of stearic acid. Paraffin is solidified in an aluminum mold after melted and mixed with stearic acid. Figure 5(b) is a plate of polyethylene. The liquid medium for paraffin and polyethylene are distilled water and silicone oil (Dow Corning Toray Silicone, SH200, kinetic viscosity: 50mm2/s), respectively.
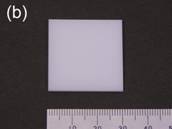
Figure 5: Materials for fabrication. (a) Paraffin in the aluminum mold. (b) Polyethylene plate.
Result & Discussion
Paraffin microstructures
We formed paraffin into pillars, oblique pillars, and lines. These structures were fabricated at the temperature of paraffin and distilled water of 40oC. Figure 6 shows the SEM micrograph of the paraffin pillars. These structures were fabricated at laser power of 5.0 W and an irradiation time of 4.0 s. The variation of the size of pillars is caused by the inhomogeneous structure of paraffin. Figure 7 shows the micrograph of paraffin pillars fabricated at laser power 5.6 W and for various irradiation times. Figure 8 shows the heights and diameters of paraffin pillars as a function of laser beam irradiation time for various laser powers. The height increases with laser irradiation time. The diameter, on the other hand, first increases and saturates for longer times, depending on the laser power. Thus, height and diameter of the pillar can be controlled by irradiation time and laser power.
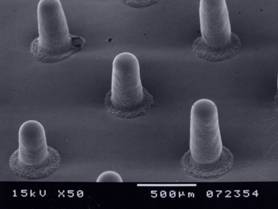
Figure 6: SEM micrograph of Paraffin pillars fabricated at laser power of 5.0 W and irradiation time of 4.0 s.
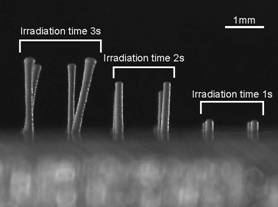
Figure 7: Micrograph of Paraffin pillars fabricated at laser power of 5.6 W and for various irradiation times.
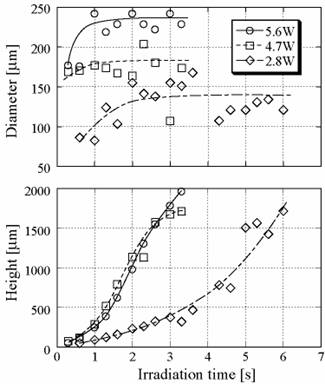
Figure 8: Diameters/heights of paraffin pillars.
Figure 9 shows the SEM micrograph of oblique pillars of paraffin which were fabricated by inclined laser irradiation. The oblique angle of pillars is 22o, which is approximately same as that of the laser in the liquid.
Figure 10 shows the SEM micrograph of line structures of paraffin. These were achieved by laser scanning with a scan speed of 200 µm/s and a laser power of 5.0 W. The height and width are respectively 240 µm and 270 µm. Both the height and the width of lines can be controlled by the scanning velocity and power of laser.
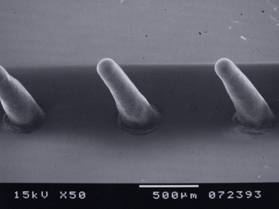
Figure 9: SEM micrograph of oblique pillars of paraffin fabricated at laser power of 5.0 W and irradiation time of 4.0 s.
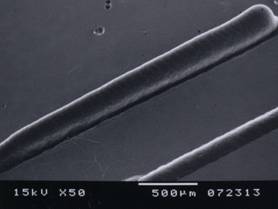
Figure 10: SEM micrograph of paraffin lines fabricated at laser scanning velocity of 200 µm/s and power of 5.0 W.
Polyethylene microstructure
Figure 11 shows the SEM micrograph of the polyethylene microstructure which was fabricated at laser power of 5.0 W and an irradiation time of 40 s and the temperature of polyethylene plate and silicone oil of 100oC. The maximum diameter and height are 450 µm and 260 µm, respectively. Compared to paraffin, the fabrication of polyethylene microstructures requires more time. In addition, it is hard to grow to a high aspect ratio. The reason for these results would be much higher viscosity of molten polyethylene than molten paraffin.
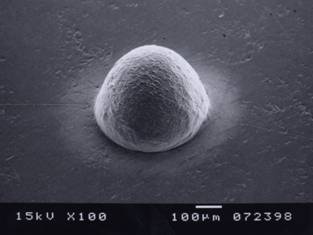
Figure 11: SEM Micrograph of a polyethylene microstructure fabricated at laser power of 5.0 W and irradiation time of 40 s.
Conclusions
We have developed a new technology to fabricate 3D convex microstructure from thermoplastic materials with laser heating. It is possible to control the size and shape of these microstructures by the laser power and irradiation time. With this method, microstructures of paraffin and polyethylene have been fabricated.
Our future work will focus on fabricating more minute and precise structures by reducing the laser spot size, and extending our research to other polymeric materials such as poly(methylmethacrylate) (PMMA) or polypropylene. Also, we aim to incorporate this technique on chemical and biological applications.
Reference
[1] N. Tsukada, T. Nakao, and T. Higuchi, "3D MICROSTRUCTURES FABRICATION OF PARAFFIN OR PLASTICS WITH LASER", Proceedings of the 18th IEEE International conference on Micro Electro Mechanical Systems (MEMS 2005), pp. 576-579 (2005/1)